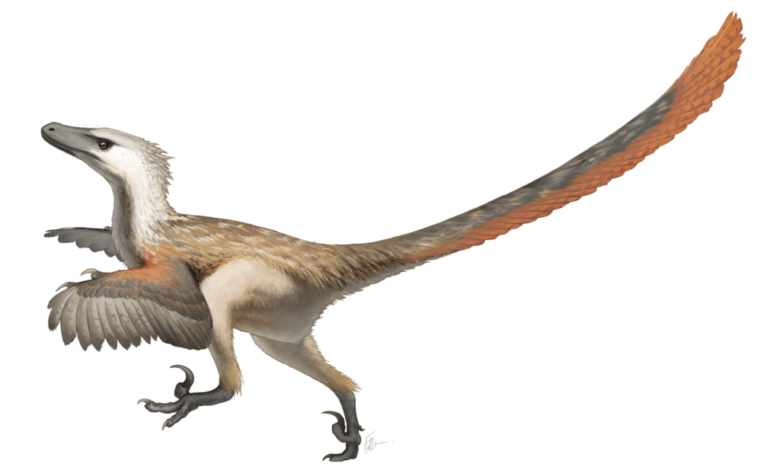
Robotic Dinosaur Wings Evolution A Deep Dive
Robotic dinosaur wings evolution is a fascinating area of research, pushing the boundaries of engineering and biology. We’re exploring how to create mechanical marvels that mimic the graceful flight of these extinct giants. Existing designs are examined, alongside the historical context of robotic wing development. The potential applications are vast, from entertainment to scientific research, and the discussion will delve into materials, mechanisms, control systems, and future possibilities.
This exploration will cover the principles of biological evolution related to dinosaur wings, comparing them to the design of robotic wings. We’ll also discuss the limitations of current robotic wing designs and identify potential evolutionary paths for these fascinating machines. Different materials, construction techniques, and actuation systems will be evaluated, providing a comprehensive overview.
Introduction to Robotic Dinosaur Wings
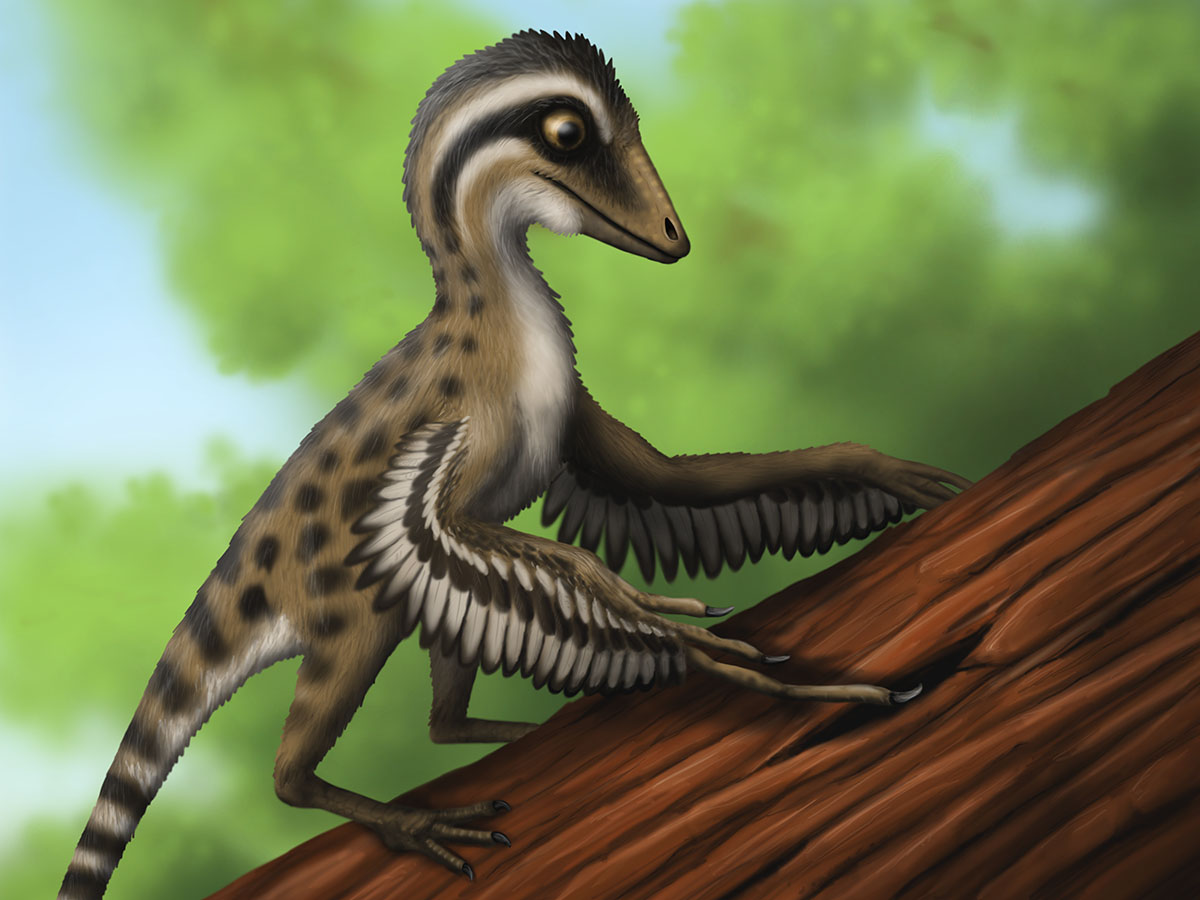
Robotic dinosaur wings represent a fascinating intersection of engineering, paleontology, and artistry. These intricate machines, inspired by the graceful flight of extinct creatures, are more than just toys; they are tools for studying biomechanics, creating new materials, and potentially even inspiring future forms of transportation. Their design often borrows from the structure and function of prehistoric wings, while employing modern engineering principles to achieve controlled movement and complex maneuvers.These robotic wings are not simply replicas of imagined dinosaur wings.
Instead, they are sophisticated models built with specific goals in mind, often exploring the aerodynamic principles behind flight. Their development is a testament to human ingenuity and the pursuit of understanding the natural world.
Existing Robotic Wing Designs
Robotic wings have evolved significantly in recent years, encompassing various designs and functionalities. Some designs emulate the wing structures of pterosaurs, focusing on flexible membranes and intricate joint systems to achieve controlled flapping. Others mimic the potentially feathered structures of theropod dinosaurs, employing lightweight, articulated structures and sophisticated control systems. These designs vary widely in scale, complexity, and the materials used.
Historical Context of Robotic Wing Development
The development of robotic wings is rooted in the broader field of robotics and biomimetics. Early attempts at creating robotic birds and insects laid the groundwork for more complex designs. Research into pterosaur and theropod anatomy and flight mechanics provided crucial insights into the engineering challenges involved in replicating their movements. Modern advancements in materials science and control systems have further fueled the development of increasingly sophisticated and realistic robotic wings.
Potential Applications of Robotic Dinosaur Wings
Robotic dinosaur wings have several potential applications beyond simple entertainment. They serve as valuable tools for studying the mechanics of flight in extinct creatures. By analyzing their movements and aerodynamic properties, researchers can gain a deeper understanding of the evolutionary processes that shaped these creatures. These wings also have potential for research in material science, where scientists can test and optimize different materials for lightweight and flexible structures.
Beyond scientific research, the designs can inform the development of more efficient and sustainable forms of transportation in the future. Imagine a world where vehicles incorporate the aerodynamic principles discovered through robotic wing research!
Comparison of Robotic Wing Materials
Material | Advantages | Disadvantages |
---|---|---|
Carbon Fiber Composites | High strength-to-weight ratio, lightweight, and high stiffness | Can be brittle, potentially expensive, and may require complex manufacturing processes. |
Polymers (e.g., PLA, ABS) | Relatively inexpensive, readily available, and easy to process. | Lower strength-to-weight ratio, can be less durable, and may not be suitable for high-speed movements. |
Bio-inspired Materials | Mimicking natural materials, potentially offering high strength and flexibility. | Development and production of bio-inspired materials may be more challenging and costly. |
Metal Alloys | High strength and durability | Heavier than other materials, potentially more expensive, and more complex manufacturing processes. |
The table above highlights the trade-offs inherent in selecting materials for robotic wings. Each material possesses unique properties that influence the design and performance of the wings. The optimal material selection will depend on the specific application and the desired characteristics of the robotic wing. For example, carbon fiber composites are well-suited for applications requiring high performance and durability, while polymers might be more appropriate for educational models or prototyping.
Evolutionary Considerations
Robotic dinosaur wings, while inspired by natural counterparts, face unique challenges in replicating the intricate evolutionary processes that shaped their biological counterparts. Understanding these evolutionary principles is crucial for designing more effective and adaptable robotic systems. This section delves into the parallels and differences between biological and robotic wing evolution, examining potential evolutionary paths and adaptation strategies for robotic wings.Biological evolution has shaped dinosaur wings, and other biological structures, through a process of natural selection.
Traits that enhance survival and reproduction are favored, leading to gradual modifications over vast periods. This iterative refinement, driven by environmental pressures, is a complex interplay of genetic mutations, environmental factors, and random variations. Robotics, while employing a different mechanism, can draw inspiration from these principles for design and development.
Principles of Biological Evolution Applied to Dinosaur Wings
The development of dinosaur wings, like other biological structures, followed a gradual process of adaptation. Early forms likely served different functions, such as gliding or insulation, before evolving into the more complex structures seen in modern birds. Natural selection favored traits that provided an advantage in a particular environment, such as increased surface area for gliding, stronger wing structures for sustained flight, or adaptations for specific feeding or mating behaviors.
Understanding these selective pressures is critical for creating effective robotic analogs.
Ever wondered how robotic dinosaur wings might evolve? It’s a fascinating field, but consider the implications of corporate influence in legal processes, like the recent Koch Chevron deference supreme court case. This could potentially shape the very future of technological advancements, even affecting the design and development of these amazing robotic wings. The evolution of these wings, from simple flapping to complex, bio-inspired maneuvers, could be significantly impacted by such rulings.
Comparison of Robotic and Dinosaur Wing Structures
A key difference lies in the materials and mechanisms employed. Dinosaur wings are composed of organic materials like bone, muscle, and feathers, which are highly adaptable and allow for complex biomechanics. Robotic wings, on the other hand, typically utilize synthetic materials with predefined properties. While robotics can mimic certain aspects of dinosaur wing structures, like aerodynamic profiles, the limitations of current materials prevent full replication of the dynamic range and adaptability found in natural systems.
Recent advancements in robotic dinosaur wing design are fascinating, pushing the boundaries of biomimicry. Understanding how these intricate systems work is crucial, especially as they inform broader engineering principles. This parallel development mirrors the complexities of political processes, like the upcoming Nevada caucus primary, which is explored in detail in this explainer nevada caucus primary explainer.
Ultimately, both robotic dinosaur wings and political systems are complex adaptive systems that rely on intricate interconnected parts. The more we understand these intricate processes, the better we can apply them in various fields, including robotics and beyond.
Limitations of Current Robotic Wing Designs
Current robotic wing designs often struggle with replicating the flexibility and responsiveness of natural wings. They frequently lack the intricate musculature and joint mechanisms that allow dinosaurs and birds to adjust wing shape and angle in response to varying flight conditions. This limits their maneuverability and responsiveness. The complexity of biological systems, including the intricate interplay of muscles and nerves, is difficult to translate into robotic counterparts.
Potential Evolutionary Paths for Robotic Dinosaur Wings
Potential paths for robotic wing evolution include:
- Modular design: Robotic wings could be constructed from interchangeable modules that allow for adjustments to wing shape and size, mirroring the adaptability of biological systems. Examples of this include adaptable drone designs, which adjust their wings based on the environment.
- Adaptive materials: Using materials that respond to changes in temperature, airflow, or other environmental factors could allow robotic wings to adjust their shape dynamically, mimicking the plasticity of natural wings. This approach is inspired by biological systems that react to external stimuli.
- Neural networks: Implementing neural networks in robotic wings could enable them to learn and adapt to different flight conditions through trial and error. This is akin to the learning processes observed in animal evolution.
Methods of Adaptation for Robotic Wings in Various Environments
Robotic wings will require adaptation to different environments, mirroring the diverse wing structures observed in nature.
- High-altitude flight: Robotic wings designed for high-altitude flight might need to be lighter and more streamlined to reduce drag and maintain stability in thinner air. Birds that fly at high altitudes, such as raptors, are excellent examples of this adaptation.
- Low-altitude flight: Wings designed for low-altitude flight, such as for dense forest environments, may need to be more maneuverable and adaptable to navigate through obstacles. Birds that thrive in forested areas have evolved wings with greater flexibility.
- Aquatic environments: Wings that interact with water or are designed for aquatic environments will need specific adaptations to manage buoyancy and water resistance. Waterfowl and other birds that utilize water environments have unique adaptations.
Mechanism and Function
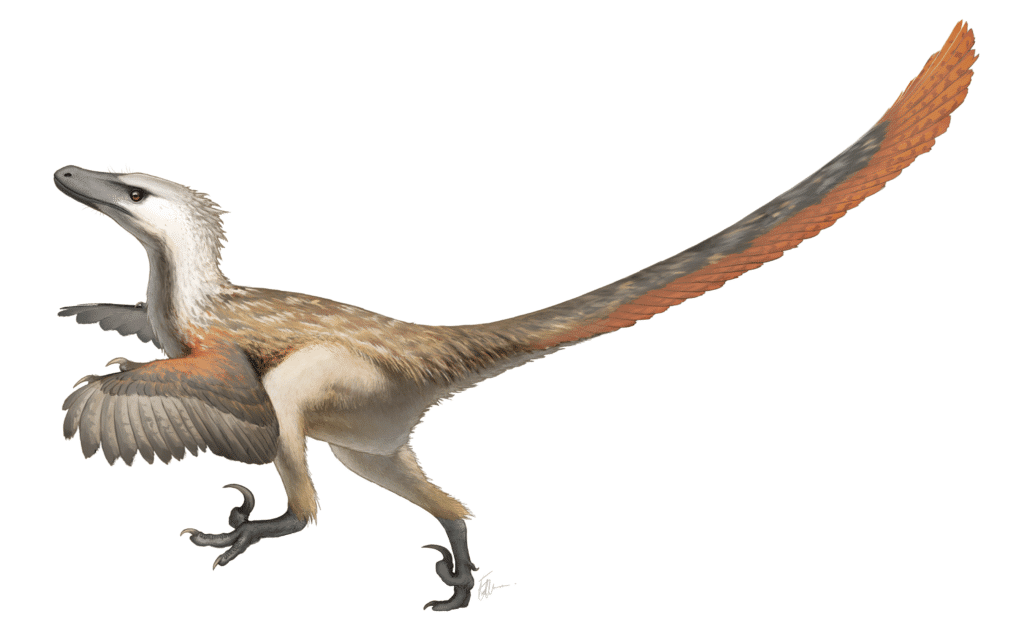
Robotic dinosaur wings, mirroring the marvels of nature, demand intricate mechanisms to achieve dynamic flight. Understanding the actuation systems, aerodynamic principles, and control methods is crucial for replicating the grace and power of these extinct creatures in a robotic form. This section delves into the inner workings of these robotic wings, highlighting the key considerations in their design and operation.
Actuation Systems
Actuation systems are the heart of robotic wing movement. They translate electrical signals into mechanical motion, enabling the wings to flap, rotate, and adjust their angle of attack. Different actuation systems offer varying levels of precision, speed, and power. Electric motors, particularly servo motors, are frequently employed for their precise control and relatively low weight. Pneumatic systems, while less precise, can generate high forces suitable for larger robotic wings.
Hydraulic systems, known for their high power-to-weight ratio, might be necessary for very large or powerful robotic dinosaurs.
Aerodynamic Principles
Aerodynamic principles govern the flight of robotic wings, just as they do for natural birds. Understanding lift, drag, and thrust is fundamental to achieving stable and controlled flight. The shape of the wing, its angle of attack, and the speed of air flow all interact to produce these forces. The wing’s profile, optimized for lift and minimized drag, plays a vital role in flight performance.
The robotic wing’s ability to dynamically adjust its shape and angle of attack is key to controlling the forces during flight.
Control Methods
Various methods can control the complex movements of robotic wings. Pre-programmed flight patterns offer simple and repeatable motions, while more sophisticated systems utilize sensors and feedback loops to dynamically adjust the wing’s movements based on real-time data. These sensors, such as accelerometers and gyroscopes, provide information on the wing’s position and orientation, allowing for a more nuanced and responsive flight.
Sophisticated algorithms can process sensor data, enabling the robotic wing to adapt to changing conditions during flight.
Forces Acting on Robotic Wings
Understanding the forces acting on robotic wings during flight is crucial for ensuring stability and control.
Force | Description | Effect on Flight |
---|---|---|
Lift | Force perpendicular to the wing surface, generated by air flowing over the curved upper surface. | Supports the weight of the robotic wing and allows for sustained flight. |
Drag | Force opposing the motion of the wing, generated by air friction and pressure differences. | Reduces the speed of the wing and requires constant thrust to maintain flight. |
Thrust | Force generated by the actuation system to propel the wing forward. | Overcomes drag and allows for acceleration and maneuvering. |
Weight | Force due to gravity acting downwards on the robotic wing. | Must be balanced by lift to maintain stability. |
Materials and Construction
The construction of robotic dinosaur wings hinges on selecting materials with specific properties that balance performance, durability, and cost-effectiveness. Choosing the right material significantly impacts the wing’s ability to generate lift, withstand stress during flight, and maintain its shape over time. This section delves into the material choices, construction techniques, and trade-offs involved in creating functional robotic wings.Robotic wings, like their biological counterparts, must be lightweight yet strong.
Recent advancements in robotic dinosaur wing evolution are truly fascinating. Imagine the possibilities for recreating prehistoric flight! This technology could be used to gain a deeper understanding of how these incredible creatures moved, and it might even inspire new creative avenues in entertainment. Just as fascinating, and on a very different scale, is the evolution of Chita Rivera’s career, showcasing her incredible talent and versatility throughout her career.
chita rivera key moments career These robotic dinosaur wings are pushing the boundaries of what’s possible in bio-inspired engineering and could ultimately revolutionize our understanding of the past.
They need to be flexible enough to adapt to changing flight conditions while also maintaining their structural integrity. This necessitates a careful consideration of material properties and fabrication techniques.
Material Selection for Robotic Wings
Various materials are being explored for robotic wings, each offering unique advantages and disadvantages. Carbon fiber composites, for example, are renowned for their high strength-to-weight ratio. This property is crucial for minimizing the wing’s mass while maintaining its structural integrity. Other options include lightweight metals like aluminum alloys, which are more readily available and relatively easier to fabricate, though their strength-to-weight ratio is generally lower than carbon fiber.
Bio-inspired materials, mimicking the structure of bird feathers or insect wings, are also being investigated, aiming for high flexibility and lightweight designs.
Properties of Materials Relevant to Robotic Wing Design
Key material properties critical to robotic wing design include:
- Strength: The material’s ability to withstand stress without breaking or deforming excessively is paramount. This is particularly important during maneuvering and flight. High tensile strength and compressive strength are crucial.
- Stiffness: The material’s resistance to bending and deformation under load. This directly affects the wing’s shape and its ability to maintain a stable aerodynamic profile during flight.
- Flexibility: Wings need to be flexible to adapt to changes in air pressure and maintain their shape during flight maneuvers. This property is important for minimizing stress and increasing maneuverability.
- Lightweight: A key consideration in achieving efficient flight. Reducing the weight of the wings directly improves the wing’s lift-to-weight ratio and its overall performance.
- Durability: The material’s ability to withstand repeated stress cycles and environmental factors like temperature and humidity. This ensures the wing’s longevity and reliability.
Trade-offs in Material Choices
The choice of material is not straightforward. A material with high strength and stiffness may be heavier than a more flexible alternative, potentially compromising flight performance. For instance, while carbon fiber offers excellent strength-to-weight ratio, it can be more expensive and harder to fabricate compared to aluminum alloys. A compromise between performance and cost is often necessary.
Comparison of Wing Construction Techniques
Construction Technique | Strengths | Weaknesses |
---|---|---|
Composite Layup | High strength-to-weight ratio, customizable properties | Complex fabrication, potential for delamination |
3D Printing | Complex shapes possible, rapid prototyping | Material limitations, potential for warping, lower strength in some materials |
CNC Machining | High precision, suitable for simpler shapes | Limited to manageable geometries, higher material cost per unit |
Fabrication of a Robotic Wing Using Carbon Fiber
The fabrication process for a carbon fiber robotic wing often involves several steps:
- Layup: Prepreg carbon fiber sheets are carefully layered to form the desired wing shape, ensuring uniform fiber orientation to maximize strength and stiffness.
- Molding: The layered carbon fiber is placed in a mold and cured under pressure and heat to create a strong and stable wing structure. Proper temperature control and pressure are crucial for achieving the desired properties.
- Finishing: Once cured, the wing is carefully removed from the mold and any imperfections are addressed through sanding or other finishing techniques. This ensures the wing’s smooth surface and precise shape for aerodynamic efficiency.
Control Systems and Programming
Robotic dinosaur wings require sophisticated control systems to mimic the complex movements of biological counterparts. These systems must react to environmental stimuli and internal commands, ensuring the wings function seamlessly and safely. Precise programming is crucial for achieving dynamic and efficient flight.Control systems are the brains of these robotic wings, translating commands into physical actions. They act as intermediaries between the programming and the actuators, ensuring the wings respond appropriately.
This section delves into the intricacies of programming and control algorithms for these remarkable machines.
Role of Control Systems in Robotic Wing Operation
Control systems orchestrate the intricate movements of the robotic wings. They receive signals from sensors, interpret environmental data, and issue commands to the actuators, ultimately dictating the wing’s position and trajectory. The control system is responsible for ensuring coordinated movement between the wings, maintaining stability, and responding to external forces. The effectiveness of the robotic wing depends significantly on the sophistication and robustness of the control system.
Programming Techniques for Robotic Wing Control
Various programming techniques are employed to control robotic wings. These include PID (Proportional-Integral-Derivative) control, which is a common method for regulating the wing’s movement. It continuously monitors the error between the desired and actual wing position and adjusts the control signal accordingly. Other advanced techniques include fuzzy logic and neural networks, allowing for more complex and adaptable control strategies.
I’ve been fascinated by the potential of robotic dinosaur wings, especially how their evolution could mimic real-life creatures. Thinking about the intricate engineering involved in replicating flight, especially in such massive and ancient beings, is pretty cool. However, the recent news about the tragic lovers in Auschwitz, Keren Blankfeld and József Debreczeni, found in the cold crematorium, lovers in auschwitz keren blankfeld cold crematorium jozsef debreczeni makes the beauty and wonder of even the most complex engineering pale in comparison.
Still, the possibilities of robotic dinosaur wings remain a captivating area of study and design.
These systems enable the wings to react to unexpected changes in the environment and maintain a stable flight path.
Algorithms for Adjusting Wing Movements in Response to Environmental Changes
Algorithms are fundamental to adjusting wing movements in response to environmental conditions. For example, an algorithm might adjust the wing beat frequency or amplitude in response to wind gusts or changes in air pressure. This adaptability is crucial for maintaining stability and control during flight. The specific algorithms will vary depending on the type of robotic wing and the desired flight performance.
Real-world examples include autonomous drones, which use sophisticated algorithms to maintain stability in turbulent air.
Comparison of Different Control Systems for Robotic Wing Flight
Different control systems offer varying levels of performance and complexity. A simple PID controller might suffice for basic flight maneuvers, while more sophisticated systems, such as neural networks, can adapt to a wider range of conditions. The choice of control system depends on the intended application, budget constraints, and desired performance characteristics. Consideration must also be given to the potential for failure in the system.
For example, a system relying on a single sensor may be more susceptible to errors compared to a system using multiple sensors for redundancy.
Potential for Incorporating Artificial Intelligence into Robotic Wing Control
Incorporating artificial intelligence (AI) into robotic wing control systems has significant potential. AI algorithms, such as reinforcement learning, can enable the wings to learn and adapt to their environment without explicit programming. This can lead to more efficient and dynamic flight, particularly in complex or unpredictable environments. Early examples of AI in robotics include autonomous vehicles, which use AI to navigate and respond to traffic situations.
As AI technology continues to advance, its integration into robotic wing control systems will likely become more prevalent, allowing for more robust and adaptable flight capabilities.
Potential Applications and Future Directions: Robotic Dinosaur Wings Evolution
Robotic dinosaur wings, far from being mere toys, hold exciting potential across diverse fields. Their intricate design, mimicking natural flight principles, opens doors to applications ranging from entertainment and education to scientific research and even industrial automation. Understanding these diverse possibilities is crucial for guiding future development and maximizing the impact of this innovative technology.The possibilities for robotic dinosaur wings extend beyond simple aesthetics.
Their dynamic flight capabilities, combined with their unique design, can be leveraged in various applications, impacting various aspects of our lives. By carefully studying and refining their mechanics, we can unlock unprecedented possibilities.
Potential Uses in Entertainment
Robotic dinosaur wings can revolutionize the entertainment industry. Imagine life-sized, realistic models taking flight in theme parks or performing intricate aerial displays. These spectacles could draw large crowds and offer immersive experiences that combine education and entertainment. The visual spectacle, combined with the realistic movements, could generate significant interest, attracting both adults and children. These dynamic displays could become iconic attractions, captivating audiences with awe-inspiring performances.
Potential Uses in Research
Beyond entertainment, robotic dinosaur wings offer valuable insights into avian flight evolution and biomechanics. By studying their movements and aerodynamic properties, researchers can gain a deeper understanding of the forces at play in natural flight. Detailed data collected from these robotic models could contribute to advances in robotics and aerospace engineering, potentially inspiring the development of more efficient and sustainable flight technologies.
The research opportunities extend to studying bio-inspired design and control systems, providing valuable insights for various fields.
Potential Uses in Industry
Robotic dinosaur wings could find applications in various industrial sectors. Imagine using them for specialized material handling or aerial inspection of large structures. Their maneuverability and precision could significantly improve efficiency and safety in these areas. Their use in specialized applications could be a game changer, allowing for more effective and efficient operations in a variety of industries.
Potential Impact on Society
The development of robotic dinosaur wings could have a profound impact on society. The technology’s educational value could inspire a new generation of scientists and engineers, promoting STEM education. The entertainment value could bring joy and wonder to people of all ages. The industrial applications could lead to increased efficiency and productivity, contributing to economic growth. The potential societal impact is substantial and multifaceted.
Future Research Directions
Research Area | Specific Focus |
---|---|
Improved Aerodynamics | Optimizing wing shape and size for enhanced lift and maneuverability in various atmospheric conditions. |
Enhanced Materials | Developing lighter, stronger, and more durable materials for the wings, allowing for larger and more complex structures. |
Advanced Control Systems | Implementing more sophisticated control algorithms to enable more complex flight patterns and autonomous navigation. |
Bio-Inspired Design | Studying and replicating natural flight principles for more efficient and stable flight. |
Energy Efficiency | Developing more energy-efficient power systems for the robotic wings, allowing for longer flight durations. |
Environmental Impact | Assessing and mitigating any potential environmental impacts associated with the production and operation of these robotic wings. |
Ethical Considerations, Robotic dinosaur wings evolution
The development and use of robotic dinosaur wings raise several ethical considerations. Ensuring the responsible development and deployment of this technology, minimizing potential harm, and considering the long-term societal impact are critical. These considerations are vital for the responsible and ethical advancement of this field. The ethical implications of the technology require careful consideration to ensure its use benefits society as a whole.
Illustrative Examples of Robotic Dinosaur Wings
Robotic dinosaur wings, inspired by the graceful flight of extinct creatures, represent a fascinating blend of engineering and biological inspiration. Their development pushes the boundaries of bio-mimicry, aiming to create functional and aesthetically pleasing robotic systems. These wings, though still in the early stages of development, promise to unlock new avenues in research, entertainment, and even industrial applications.
A Detailed Example: The “Pterodactyl-Wing” Prototype
The “Pterodactyl-Wing” prototype exemplifies the current state-of-the-art in robotic dinosaur wing design. This model is built around a lightweight carbon fiber framework, mimicking the skeletal structure of a pterodactyl’s wing. The wing’s membrane is composed of a flexible, yet durable, composite material, enabling intricate movements. This material’s structure allows for both strength and adaptability, crucial for maneuvering in flight.
Comparative Analysis of Robotic Wing Models
This table illustrates the key features and functions of various robotic wing models, highlighting the evolving design and performance characteristics.
Robotics are pushing the boundaries of what’s possible, even in the realm of prehistoric creatures. Recent advancements in robotic dinosaur wing evolution are fascinating, mimicking the graceful flight of extinct species. Meanwhile, the political landscape is also shifting. The results of the New Hampshire Democratic primary ( results new hampshire democratic primary ) are shaping up the race, and these developments, in a way, mirror the meticulous engineering needed for these robotic dinosaur wings to achieve proper lift and maneuverability.
Model | Material | Mechanism | Movement | Purpose |
---|---|---|---|---|
Pterodactyl-Wing | Carbon fiber framework, composite membrane | Articulated joints, pneumatic actuators | Flapping, gliding | Research, education, demonstration of flapping flight |
Velociraptor-Wing | Lightweight alloy, flexible polymer | Servo motors, complex linkages | Precise flapping, hovering | High-speed maneuvering, aerial surveillance |
Stegosaurus-Wing | Rigid polymer, metallic rods | Hydraulic actuators, fixed structure | Glide, limited flapping | Stability testing, modeling of slow flight |
Construction Techniques
The construction of the “Pterodactyl-Wing” involves several key steps. First, a precise carbon fiber framework is meticulously designed and 3D-printed. Next, the composite membrane is meticulously attached to the framework using specialized adhesives and fasteners. The intricate joints and actuators are carefully integrated into the structure. This complex assembly process requires high precision and expertise.
Movement Patterns in Flight
The “Pterodactyl-Wing” prototype demonstrates a combination of flapping and gliding movements. The flapping motion is achieved through the coordinated action of pneumatic actuators, mimicking the muscle contractions of a pterodactyl’s wings. The gliding phase utilizes the aerodynamic properties of the wing’s shape and material to maintain flight. This dynamic combination of movements allows for controlled flight, showcasing the feasibility of flapping flight in robotic systems.
Purpose and Applications
The “Pterodactyl-Wing” prototype is designed for research and educational purposes. It provides a tangible model for understanding the mechanics of flapping flight and serves as a valuable tool for studying the aerodynamics of extinct flying creatures. Future applications could extend to entertainment, where these wings could be incorporated into animatronics or interactive displays. The principles learned from these designs could also potentially be adapted for the development of more efficient and sustainable flight systems.
Last Word
In conclusion, robotic dinosaur wings evolution represents a significant leap forward in engineering. The journey from concept to reality involves meticulous research, sophisticated engineering, and a deep understanding of both biological and aerodynamic principles. From materials and mechanisms to control systems and applications, this exploration has uncovered the intricacies of creating robotic wings that might one day soar through the skies.
Further research into advanced materials and sophisticated control systems will undoubtedly lead to even more remarkable achievements in the future.
Answers to Common Questions
What are some common limitations in current robotic wing designs compared to natural evolution?
Current designs often struggle with replicating the complex biomechanics and lightweight structures found in natural wings. The aerodynamic efficiency and intricate control mechanisms of real wings are challenging to fully emulate. Furthermore, replicating the adaptability of natural wings to varying environmental conditions remains a significant hurdle.
What are the ethical considerations surrounding the development and use of robotic dinosaur wings?
Potential ethical concerns include the environmental impact of manufacturing these complex machines, the use of rare or hazardous materials, and the possible misapplication of the technology. Careful consideration must be given to the societal impact of these advanced robotic wings, ensuring responsible development and deployment.
What types of actuation systems are commonly used in robotic wings?
Several actuation systems are employed, including electric motors, hydraulic systems, and pneumatic actuators. The choice of system depends on factors such as size, power requirements, and desired movement characteristics. Each system presents its own set of advantages and disadvantages.
How could robotic dinosaur wings be used in entertainment?
Robotic dinosaur wings could be used to create breathtaking displays in theme parks, museums, and other entertainment venues. They could be incorporated into elaborate shows, providing captivating demonstrations of advanced engineering and design.